EAM Starting Grants
Support of young scientists
EAM’s major measurement to support scientific independence of postdoctoral researchers and junior group leaders have been the EAM Starting Grants. A total of 13 grants have been awarded between 2011-2015. In 2020, the funding scheme has been renewed and since then, EAM has granted 11 more EAM Starting Grants.
EAM Starting Grant – a success story
With the encouragement of inventive and risky projects, EAM supported young researchers in their individual career – with success. Eight of the awardees could transfer their research into ERC grant projects so far.
EAM Starting Grants 2023/2024
Dr. Carlos Bassani - Kinetic Pathways of Lower Symmetry Nanocrystal Habits
Kinetic Pathways of Lower Symmetry Nanocrystal Habits
Dr. Carlos Lange Bassani, 32
Humboldt Postdoctoral Fellow at the Institute for Multiscale Simulation
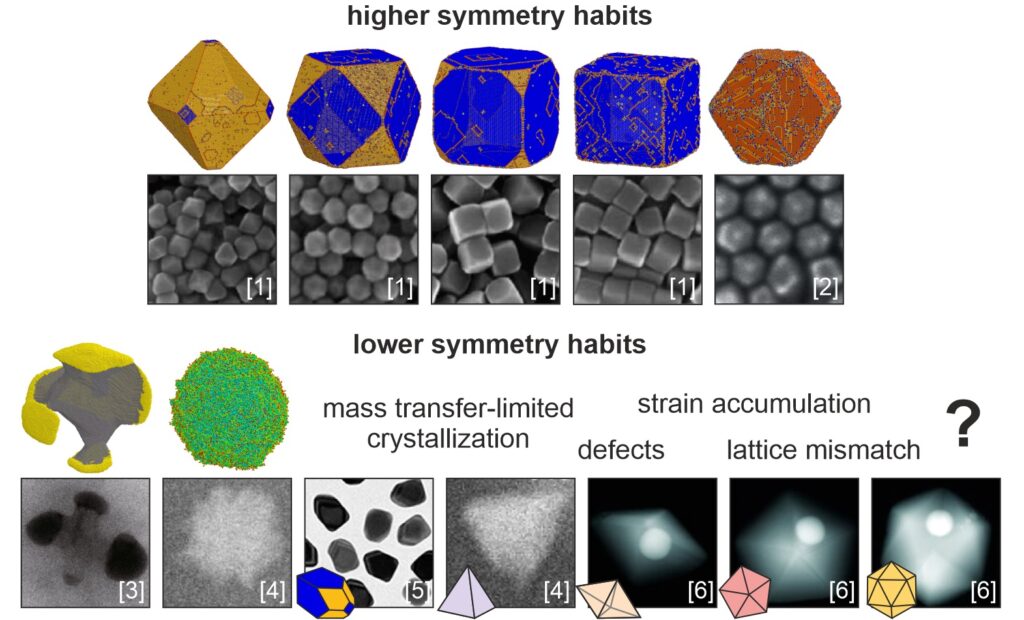
The possibility of assembling nanocrystals (NCs) into superlattices represents a new way of tuning material properties. The variety of NC habits and the possibility of their surface functionalization with shorter or longer-range interactions makes it possible to trespass the barriers so far believed in chemistry. Experimentalists thrived in forming NCs with controlled size and shape and assembling them into different superstructures. Their functionality nevertheless depends on the precise control of NC habits and superstructure formation, as well as on the electronic coupling between NCs – that is, an inherently multiscale process -, but multiscale models nevertheless did not keep the pace of the recent advances in the field. This project tackles the upscaling of atomic to realistic-sized NC scales formed of 10s-of-millions atoms via a new rejection-free Kinetic Monte Carlo method based on the semi-Gibbs ensemble. Of special attention is the role of strain accumulation via defects, lattice mismatch, and geometric frustration in changing the dynamic pathway of crystalline growth, thus kinetically entrapping NCs into lower symmetry habits – that is, shapes that do not comply with the underlying symmetry of the crystalline structure. The coupling with reactor scales (environment) to understand mass transfer-limited crystallization is also pivotal to predicting the yield of denser NC populations. Bridging the multiscale understanding from atom to NC to the environment will make it possible to optimize NC synthesis conditions and potentially lead to discovering new NC habits.
Contact: carlos.bassani@fau.de
References of microscope image: [1] Xia et al, J. Am. Chem. Soc. 2012, 134 (3), 1793–1801, [2] Ahn et al, J. Mat. Chem. C 2013, 1, 6861, [3] Chen et al, Nature Comm. 2020, 11 (1), 3041, [4] Sun et al, ACS Nano 2021, 15 (10), 15953–15961, [5] Xia and Xia, Nano Lett. 2012, 12 (11), 6038–6042, [6] Langille et al, Science 2012, 337 (6097), 954–957.
Prof. Dr. Siow Woon Ng - Probing Electrochemical Atomic Layer Deposition
Probing Electrochemical Atomic Layer Deposition
Prof. Dr. Siow Woon Ng, 35
Junior Professor for Physical Chemistry at Interfaces
Atomic layer deposition (ALD) remains as a leading thin film deposition technique. Its signature characteristics of self-limiting surface reactions offers exceptionally well controlled growth: Atomic precision of layer thickness, high-quality homogeneous films, and conformal coverage. ALD is highly favored for preparing functional, seeding or passivation layers, for a wide range of materials in micro- and opto-electronics, photovoltaics, catalysis, batteries, sensors, and bio-medical industries. Electrochemical atomic layer deposition (E-ALD) combines the strength of two liquid phase deposition techniques: electrodeposition and solution ALD. E-ALD explores electrochemical control of chemical interactions with the substrate in liquid environment, while preserving the self-limiting surface reaction. This approach broadens the options of precursor and allows the deposition at more reasonable conditions compared to conventional ALD. This project aims to investigate electrochemical surface-limited reactions, observe the nucleation and growth of thin films using scanning probe electrochemical methods, and ultimately striving for atomic-level control of deposition.
Contact: siowwoon.ng@fau.de
Dr.-Ing. Christopher H. Zenk - SPACE - Superior Performance through Accelerated Composition Exploration
SPACE – Superior Performance through Accelerated Composition Exploration
Dr.-Ing. Christopher H. Zenk, 37
Group Leader High Performance Alloys
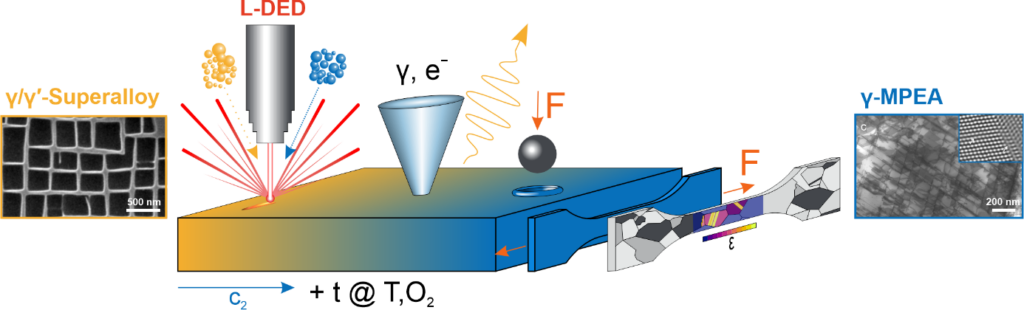
Power generation and propulsion demand cutting-edge alloys operating in extreme conditions. Superalloys, key to efficient gas turbines and aerospace engines, not only require an excellent high-temperature creep strength, but also exceptional resistance to oxidation and corrosion. However, their significance expands beyond current technologies, as novel high-performance alloy systems are also key in clean concepts like hydrogen-fueled aircraft engines and high-temperature solid-state oxide fuel cells. Additionally, in concentrated solar power plants and nuclear fission reactors, steam turbines are the key component to convert the generated heat into usable electricity. Even in future nuclear fusion reactors, steam turbines are envisaged to extract the massive thermal energy of the plasma and its efficiency increases with the operating temperature, which in turn is limited by the materials’ high temperature strength in presence of steam.
SPACE – “Superior Performance through Accelerated Composition Exploration” aims to revolutionize alloy discovery, development, and characterization. Unlike traditional, time-consuming methods, SPACE employs a combinatorial approach, producing and testing compositionally graded bulk specimens. Through Laser-Based
Directed Energy Deposition (L-DED) in inert Ar-atmosphere and high-throughput characterization, SPACE will assess phase stability, microstructure, diffusion, corrosion resistance, and mechanical properties on the meso-scale as a function of composition, atmosphere, temperature, and time.
Contact: christopher.zenk@fau.de
EAM Starting Grants 2022/2023
Prof. Dr. Philipp Pelz - Scattering-Enhanced Electron Microscopy: boosting resolution und throughput by imaging through scattering materials
Scattering-Enhanced Electron Microscopy: boosting resolution and throughput by imaging through scattering materials
Prof. Dr. Philipp Pelz, 37
Tenure-Track Professor at the Institute of Micro- and Nanostructure Research
State-of-the-art three-dimensional electron microscopy provides atomic-level detail of ultrathin nanoscale materials, enabling materials modeling and property prediction from first principles. However, scale-bridging three-dimensional imaging with atomic-level detail has proven challenging due to instrument instabilities and hard-to-interpret multiple-scattering effects.
Strong coherent scattering of electrons by materials, here a Gold nanoparticle below a single-crystalline substrate,
will be utilized in the ScatterEM project to enhance and accelerate electron microscopy methods.
Copyright: Philipp Pelz
This project targets these limitations by co-designing strongly scattering crystalline substrates and interference-based computational microscopy schemes that solve the material structure under multiple scattering conditions. Specifically, this project will enhance the 3D atomic resolution imaging throughput of crystalline materials by more than 50-fold by eliminating the need to sample the atomic lattice through interference-based computational imaging. Combined with tomographic data acquisition, this will enable atomic-resolution 3D imaging of thin films with micrometers of field of view and micro- to atomic scale zoom.
Contact: Prof. Dr. Philipp Pelz, philipp.pelz@fau.de
EAM Starting Grants 2021/2022
Dr. Ryan Crisp - Quantum-Enhanced Spectral Technology (QuEST)
Quantum-Enhanced Spectral Technology (QuEST)
Dr. Ryan Crisp, 33
Post-Doctoral Researcher at the Chair for Chemistry of Thin Film Materials
The major scientific challenge of the current generation is curtailing the effects of climate change. Increased focus must be placed on utilizing renewables for producing fuels to achieve the EU goal of a low-carbon economy by 2050. To take advantage of renewable fuel production, we need to answer the questions: how do we drive chemical reactions that require large/concentrated amounts of energy, and do so using more clever designs? Nanomaterials enable a unique approach to these challenges, providing the ideal framework to understand the photophysics and photochemistry involved. In this project, quantum effects arising in semiconductor quantum dots (QDs) are exploited to improve the kinetics and efficiency of photochemical reactions. The work develops a new class of solar-to-fuel systems to investigate both the fundamental processes involved in photo-catalysis and to apply the discoveries to working devices. Here, we synthesize novel BaZrS3 perovskite QDs, develop new deposition methods for the QDs while tuning the energy band levels, and test the efficiency and the kinetics of the oxygen evolution reaction.
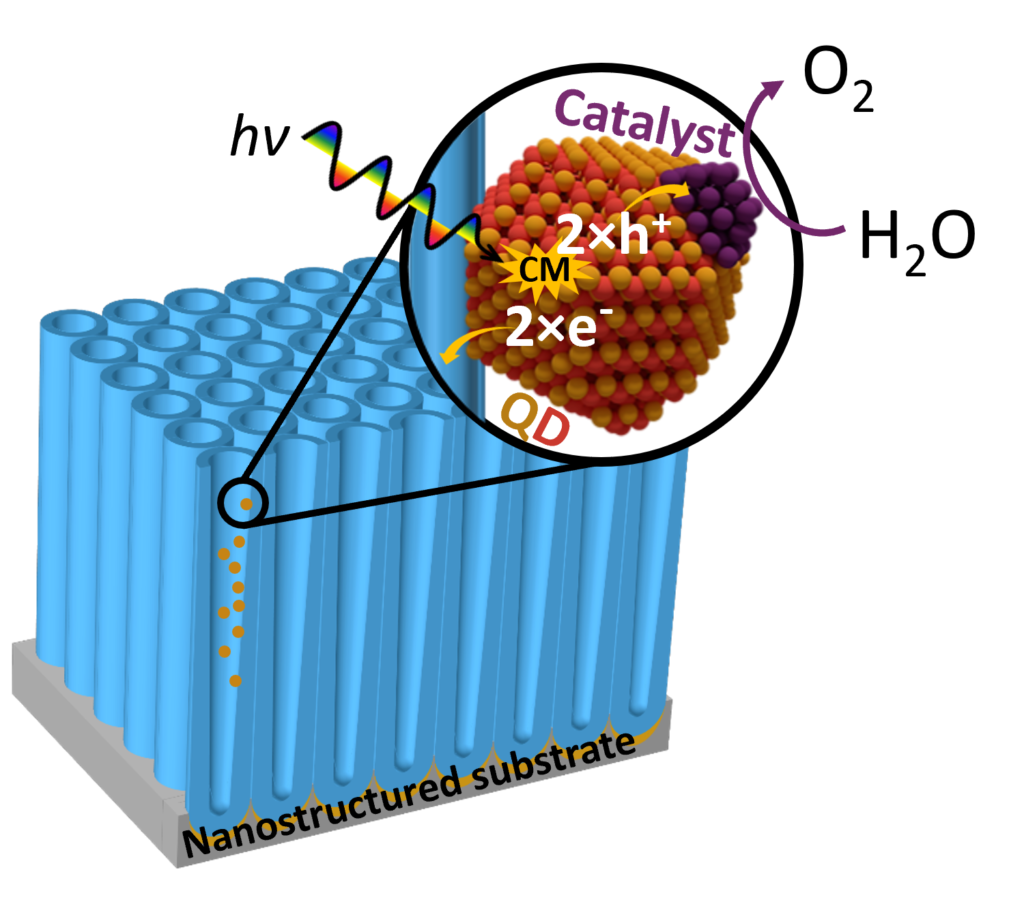
Contact: ryan.crisp@fau.de
Webpage: https://www.chemistry.nat.fau.eu/ctfm-chair/crisp-group/
Prof. Dr. Danijela Gregurec - Wireless Electrical Neuromodulation via Magnetoelectric Nanocomposites
Wireless Electrical Neuromodulation via Magnetoelectric Nanocomposites
Prof. Dr. Danijela Gregurec, 39
Juniorprofessor for Sensory Sciences
at the Chair of Aroma and Smell Research
Deep brain stimulation (DBS) is electrical stimulation that relieves motor symptoms of Parkinson’s disease (PD). In the animal models of neurological and psychiatric diseases, DBS has improved understanding of mechanisms and neural circuits underlying these disorders. DBS, however, requires permanent implantation of robust lead electrodes in deep brain structures, limiting behavioural studies in animals, and in humans, it results in mental, physical, and hardware-related unwanted effects. Considering current limitations, an imperative in the development of new wireless technologies for brain neurostimulation, and the focus of this project is on development of biocompatible and stable nanomaterials capable of remotely delivering electrical stimuli to neuronal membranes with temporal and spatial precision.
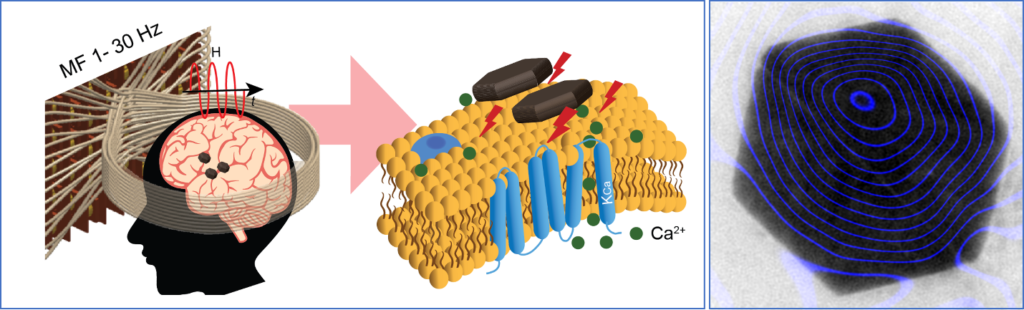
This project will design and optimize structurally and physically defined nanocomposite capable of generating local electrical fields to modulate electrical impulses of deep brain neurons under slowly varying external magnetic fields. These nanocomposites comprise biocompatible magnetostrictive core and a piezoelectric shell where actuation and polarization of nanocomposites relies on the conversion of magnetic to kinetic energy within the composite that facilitates charge separation through direct magnetoelectric effect.
Preparative methods include hydrothermal synthesis of vortex domain nanoscale mechano transducers, sputtering of magnetostrictive cores, nanosphere templating, electrospinning and electrodeposition of piezoelectric components.
These magnetoelectric materials will be evaluated and optimized for their biological applications in vitro and ultimately applied in rodent models to evaluate the potential for translational applications in treatments of neurodegenerative diseases.
Contact: danijela.gregurec@fau.de
Webpage: https://www.chemistry.nat.fau.eu/aroma-smell-research/prof-dr-danijela-gregurec/
Dr. Martin Weiser - Role of Hydrogen in High-Temperature Corrosion
ROLE OF HYDROGEN IN HIGH-TEMPERATURE CORROSION
Dr. Martin Weiser,35
Post-Doctoral Researcher at the Chair of General Materials Properties
Correlative analysis of the hydrogen effect on short-circuit diffusion during high-temperature oxidation
Solid-oxide-based cells are a key technology for efficient use of H as energy carrier. In multi-cell stacks, the usage of ferritic steels as interconnect material can reduce production costs significantly. In practice the combination of H containing gas on one side of the interconnect and a strongly oxidizing environment on the air side leads to early breakaway oxidation and premature failure of the entire device.
Transport of alloying elements and interaction with H are two essential elementary mechanisms during this unusually fast corrosion scenario and will be directly addressed in the project. The largest challenge towards the complete understanding of the so called “dual-atmosphere effect”’, is the unambiguous detection of H and its pathways during exposure of metals at high temperatures. The development of an in situ set-up in conjunction with the unique capabilities to routinely analyze oxidized samples in an atom probe with ultra low H background will reveal the effect of H at the near-atomic level. In the considered temperature range (600 – 850 °C), short-circuit diffusion, for example along grain boundaries dominates elemental transport during oxidation. Dedicated experimental strategies will therefore be used to elucidate GB diffusion (of Cr) with respect to the surrounding atmosphere. Correlative high-resolution analysis with transmission electron microscopy (TEM) and electron backscatter diffraction (EBSD) will help to gain a complete understanding of the oxidation behavior in dual atmospheres.
These new insights are the basis for the development of advanced strategies to prevent degradation of metallic materials and coating technologies that can be used for the production and storage of hydrogen as well as during energy conversion at elevated temperatures.
Contact: martin.weiser@fau.de
Webpage: https://www.ww1.tf.fau.de/
EAM Starting Grants 2020/2021
Prof. Dr. Tanja Franken - Forced surface dynamics for novel catalytic concepts
FORCED SURFACE DYNAMICS FOR NOVEL CATALYTIC CONCEPTS
Prof. Dr. Tanja Franken, 34
Junior Professor for Catalytic and Electrocatalytic Systems and Processes
at the Institute of Chemical Reaction Engineering
Exploiting the dynamic surface behavior of complex mixed metal oxides for future relevant thermo catalytic reactions
Optical change of a modified catalyst before (left) and after surface activation (right). Copyright: Prof. Dr. Tanja Franken
Avoiding the waste of energy, metals and other resources is one of the current central challenges to enable the transition to a sustainable future. In the chemical industry, sintering of catalysts significantly affects the efficiency and selectivity of processes. To overcome this issue completely new concepts need to be explored. The aim of the project is to design and stabilise highly active catalysts based on mixed metal oxides (MMOs), exploiting the dynamic behaviour of oxide surfaces near their stability limit. The activation of MMOs generates finely dispersed metal centres in close vicinity to important functional surface groups, which exhibit a high potential for high activity and selectivity in future relevant reactions for CO2 utilisation or for limiting of CH4 flaring. Examples are CO2 methanation, dry reforming of CH4 or water-gas shift reaction. The project will clarify how MMOs need to be modified in order to trigger surface dynamics and how the process needs to be operated in order to maintain highly active but transiently stable active centres. The fundamental understanding of the adaption of MMOs to generate and maintain high catalytic activity opens up completely new ways for catalyst synthesis and operation. This unconventional perspective on MMO surfaces forces a paradigm shift in the nature and capabilities of oxide based catalysts.
Contact: tanja.franken@fau.de
Webpage: https://www.crt.tf.fau.eu/forschung/arbeitsgruppen/catalytic-and-electrocatalytic-systems-and-processes/
Dr. Andreas Hutzler - Dynamic processes at atomic resolution
DYNAMIC PROCESSES AT ATOMIC RESOLUTION
Dr. Andreas Hutzler, 32
Post-Doctoral Researcher at Electron Devices (LEB)
Modeling Electron-Beam Induced Radiolysis in Liquid-Phase Electron Microscopy – Radiochemistry in LP-EM
HRTEM image series of AuNP growth and subsequent etching in 1 mmol/l HAuCl4 solution recorded at an electron dose rate of 490 e-/Å2s. Copyright: Dr. Andreas Hutzler
The objective of the project is to study the influence of the electron-beam interaction in liquid-phase electron microscopy (LP-EM) on the chemical environment of liquid specimen determining nucleation, growth, interaction and degradation of nanoparticulate systems. In order to study dynamic processes by direct observation on the nanoscale, LP-EM has been shown to be a powerful tool and an enrichment to complementary methods deploying X-ray or light scattering. For example, LP-EM was shown to be suitable for investigating the early stages of particle growth (see figure).
Although LP-EM opens up new possibilities of in situ characterization, the electron beam is known to severely influence or even trigger the observed phenomena and the environment in a liquid cell is often compared to the core of a nuclear reactor. Indeed, scientists try to prevent electron beam induced effects by using conductive membranes like graphene in combination with extremely low dose conditions like used in cryo EM. This, however, strongly limits the capabilities of modern electron microscopes in terms of temporal and spatial resolution. Furthermore, a deep understanding on the interaction of the electron beam with liquid specimen is needed in order to provide reliable predictions of non-biased processes apart from the artificial environment in LP-EM.
Hence, this project aims at verification, enhancement, and completion of existing kinetic models, which is a prerequisite for predicting chemical reactions in specimen solutions containing species beyond water. Accordingly, the results are subsequently utilized for the development of methods for measuring the actual concentrations of key species for enabling a verification of the proposed kinetic model. These studies are strongly needed for LP-EM becoming a standard characterization method in materials science, electrocatalysis and biology.
Contact: andreas.hutzler@fau.de
Webpage: https://www.leb.tf.fau.de/
Dr. Dorothea Wisser - NMR studies of carbon nitride materials for CO2 reduction
NMR STUDIES OF CARBON NITRIDE MATERIALS FOR CO2 REDUCTION
Dr. Dorothea Wisser, 33
Post-Doctoral Researcher at Erlangen Center for Interface Research and Catalysis (ECRC)
Porous Carbon Nitride and Boron Carbon Nitrides – Correlation of Structure and Performance by Solid-State NMR
Solid-state NMR at Magic Angle Spinning, carbon nitride material and CO2 reduction. Copyright: Dr. Dorothea Wisser
Carbon nitride and boron carbon nitride materials are promising new candidates for CO2 sequestration and photoreduction into methane, methanol or ethylene. The materials intrinsically contain micropores, and larger pores facilitating mass transport can be introduced during the preparation. In this project, solid-state nuclear magnetic resonance under Magic Angle Spinning (MAS NMR) will be employed to obtain an understanding of the material structures, defects and adsorption sites in molecular detail. The observation of CO2 adsorption and transformation in real time are crucial for understanding the process and for a targeted optimization of the materials, they are however challenging to implement. Therefore, in situ solid-state NMR investigation of the sorption and activation of CO2 in a continuous CO2 flow through the material will be established. For simulation of realistic industrial exhaust streams, conditions of water co-adsorption will be implemented by moist gas flows. Ultimately, light irradiation will be implemented to investigate the photoactivated CO2 reduction online under realistic reaction conditions.
Contact: dorothea.wisser@fau.de
Webpage: https://www.ecrc.fau.de/
Moritz Wolf, Ph.D. - Synergetic activation of CO2
SYNERGETIC ACTIVATION OF CO2
Moritz Wolf, Ph.D., 32
Head of Team at Helmholtz Institute Erlangen-Nürnberg for Renewable Energy (IEK-11), Forschungszentrum Jülich GmbH
Capturing synergetic effects of nitrides as carriers for catalytically active nanoparticles in situ
Schematic conversion of CO2 over nitride-supported nanoparticles (left) and transmission electron micrograph of graphitic carbon nitride (right). Copyright: Moritz Wolf, Ph.D.
The project aims to identify and investigate potentially beneficial synergies when employing nitrides as support materials in heterogeneous catalysts. For example, carbon nitride as a carrier material for catalytically active nanoparticles has a great potential for efficient (co-)activation of CO2 and can therefore strongly influence the catalytic performance. Hence, bifunctional carbon nitride carrier materials may enable multiple conversion pathways for the valorisation of CO2 in addition to the physical support of the active phase. Even though inclusion of the green-house gas CO2 as feedstock in chemical sites is an important step towards a circular economy and sustainable production of basic chemicals, carbon nitride as a carrier material in heterogeneous catalysis has been barely explored. In fact, it is primarily employed as active photocatalyst and in electrocatalysis. Other nitrides have even been shown to have intrinsic catalytic activity and may offer further increased potential for the synergistic conversion of reactants. In the project, transition metal (oxide) based catalysts will be developed using this new class of carrier materials. The catalysts will be tested in several model reactions to identify various modes of synergy between the nitrides and the active phase. The advantageous effect of the nitrides on catalysis, when compared to classical carrier materials, may be evidenced by a boost in activity, selectivity and/or stability. Possible synergies of nitrides, such as the bifunctional activation of reactants, will mainly be investigated by means of in situ and operando characterisation techniques, which are becoming increasingly important in catalyst research. Such techniques enable the monitoring of certain catalyst properties under reaction conditions that are as close as possible to the actual conditions in commercial reactors, e.g. high temperatures and elevated operating pressures. The characterisation under such working conditions requires creative approaches for coupling the analysis of the catalysts with laboratory-scale reactor set-ups. However, this is the only way to study and understand the processes at play under reaction conditions, which is of utmost importance to draw conclusions on effective synergies between support material and catalytically active nanoparticles. The combination of sophisticated in situ techniques and innovative heterogeneous (model) catalysts may elucidate fundamental questions of catalysis addressing the role of the support material.
Contact: mo.wolf@fz-juelich.de
Webpage: www.hi-ern.de/hi-ern/h2Storage/EffHydroRelease
Twitter: www.twitter.com/Wolf_Catalysis
Note: This EAM Starting Grant project will be co-financed by the Helmholtz Institute Erlangen-Nürnberg for Renewable Energy (HI ERN, IEK-11).
EAM Starting Grants 2011-2015
2015 Dr. Jakob Albert - Valuable chemicals derived from biomass
Dr. Jakob Albert , 30
Assistent Professor (Akademischer Rat) at the Institute of Chemical Reaction Engineering
Leader of working group „Biomass and Sustainable Production of Platform Chemicals”
Project: Selective oxidation of lignocellulosic biomass to formic acid and high-grade cellulose with tailor-made polyoxometalate catalysts
The main goal of the project is to design and to optimize tailor-made polyoxometalate catalysts for the stepwise oxidation of lignocellulosic biomass (i.e. wood and residues from sugar or paper industries) to produce formic acid and high-grade cellulose for further processing i.e. in white biotechnology to bio-ethanol. The results of the research project will open new horizons for the sustainable use of biogenic raw materials with regard to chemical compounds.
Biomass combines all materials of organic origin that contain carbon. Lignocellulose as a suitable biogenic substrate, seems to be a promising carbon source for the production of liquid fuels and platform chemicals. Polyoxometalates (POMs) are organo-metallic complexes of light transition metals at their highest oxidation state in combination with oxygen. These metal-oxo anion clusters can also contain multiple hetero atoms. POMs have attracted much attention in recent years due to their fascinating architectures and excellent physico-chemical properties. Moreover, many of the properties of polyoxometalate materials can be finely tuned by changing the constituents and counter cations. These features have made polyoxometalates very attractive in acid and oxidation catalysis. This novel and straightforward process aims to increase the valorization of lignocellulosic biomass in a more sustainable way. This can be achieved by producing liquid hydrogen and syngas equivalent formic acid simultaneously with high-grade cellulose as feedstock for further processing such as fermentation or derivatization. The project aims to optimize suitable POM catalyst materials and improve the related oxidation processes.
2015 Dr. Patric Müller - Simulation of reactive flows
Dr. Patric Müller, 34
Assistent Professor (Akademischer Rat) at the Institute for Multiscale Simulation
Project: Particle-based simulation of reactive flows – PARSIFAL
Reactive flows have a wide-ranging technical relevance. Their comprehension and optimization are directly linked to up-to-the-minute topics like pollution control or the manufacturing of new materials. Computer simulations have proven to be an essential tool to promote their investigation. A key ingredient of reactive flow simulation is to resolve the dynamics of the underlying fluids. These are commonly modeled by the Navier-Stokes equations which are then solved by lattice based discretization schemes. This approach has however, practical and theoretical limitations which increasingly manifest in contemporary reactive flow research. These include turbulent flow, multiphase flow, phase transitions and complicated boundaries as well as cases where Navier-Stokes modeling may be questioned at all. The aim of the project is to develop mesh free particle-based simulation methods which have the potential to circumvent several restrictions of standard reactive flow simulation. The developed methods will be tested by three prototypical and for different reasons problematic technically or medically relevant systems, these are: 1) mechanical stimulation of secondary bone healing, 2) growth of patchy coatings on nanoparticles and 3) engine combustion processes and the formation of soot. The particular specialist knowledge required to successfully investigate these highly interdisciplinary demonstrators will be provided by distinct collaborators from the EAM/FAU. Therefore the FAU provides the ideal environment to carry out the proposed project.
2015 Dr. Doris Segets - 3D-printed optoelectronic devices
Dr. Doris Segets, 33
Managing Director Erlangen Center for Functional Particle Systems
Leader of Working Group “NanoProcessing” at the Institute of Particle Technology (LFG)
Project: Tailored Colloidal Interfaces for an Efficient Processing of Multifunctional Stabilized Hybrid Materials for 3 Dimensional Printing of Optoelectronic Devices: TUNE IT³
The aim of this project is to develop a methodological toolbox for the high throughput (HT) characterization of colloidal interfaces. This is an important step towards the efficient production of multifunctional materials for the 3 dimensional printing of optoelectronic devices. To achieve this highly ambitious goal, the following strategy is implemented. First, a generally applicable approach to resolve thermodynamic and kinetic parameters of ligand binding is developed and transferred to HT experimentation. Then, methods are established to relate molecular characteristics with colloidal stability and dispersibility in different matrices. Finally, the approach will be validated by dispersing nanoparticles in polymers that are applicable to extrusion based 3D printing. ZnO quantum dots (QDs) were selected as technically relevant model system throughout all our investigations. Potential future applications are found in emerging fields like e.g. solar cells. Large impact will be created as the project enhances value chains due to multifunctional material properties and potentially leads to a breakthrough in composite materials where QDs are dispersed on a primary particle level. The project is highly interdisciplinary and connects chemical engineering and particle technology with central aspects of colloid and materials science. It originates from very fundamental questions but eventually bridges the gap to macroscopic product properties.